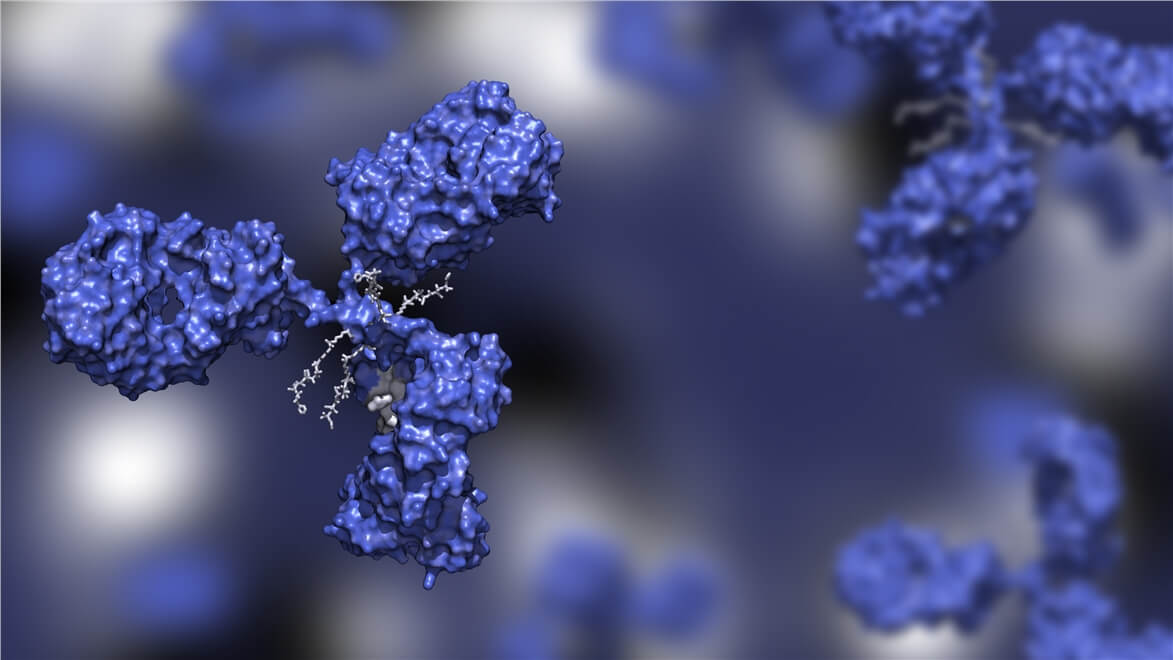
28
AugustThe Background of Circular RNA Synthesis
In recent years, nucleic acid therapy involving various types of ribonucleic acids (RNAs), including messenger RNA (mRNA), small interfering RNA (siRNA), and microRNA (miRNA), has rapidly emerged as a new cornerstone of modern medicine. Among them, the mRNA-based vaccines developed, particularly the COVID-19 vaccine, have played a significant role in combating the novel coronavirus (SARS-CoV-2) responsible for severe acute respiratory syndrome. The remarkable speed of sequence design and the ease of large-scale production associated with mRNA-based technologies have provided novel possibilities in addressing urgent and ever-evolving medical crises.
In the present context of successful mRNA drugs, the further synthesis of linear mRNA into circular RNA (circRNA) through end-to-end connections to enhance mRNA stability and prolong protein translation time has emerged as a new hot topic in the field of mRNA therapy.
In simple terms, circular RNA is a closed single-stranded RNA molecule formed through covalent linkage at its ends. It can maintain stability without the common 5' cap and 3'-poly(A) tail found in mRNA, owing to its covalently closed structure that impedes degradation by nucleases. Naturally occurring circular RNAs in organisms mostly arise from precursor mRNAs (pre-mRNA) through a process known as back-splicing. These circular RNAs are distributed in the cytoplasm and exhibit half-lives ranging from 18.8-23.7 hours, significantly longer than their linear homologs which have half-lives of only 4.0-7.4 hours.
Although the majority of natural circular RNAs are non-coding RNAs, meaning they cannot be translated into proteins, research has shown that artificially synthesized circular RNAs with internal ribosome entry site (IRES) sequences can be translated both in vivo and in vitro. The investigation of the translation mechanisms of circular RNAs in both cellular and extracellular contexts, along with the advancement of techniques for synthesizing circular RNAs in these environments, has greatly facilitated the progress of the circular RNA therapy field.
Circular RNA Synthesis Method In Vitro
Chemical Synthesis of Circular RNA
Currently, the chemical synthesis of circular RNA primarily relies on phosphoramidite chemistry and solid-phase synthesis, using naturally occurring nucleoside triphosphate derivatives as building blocks. These derivatives replace the reactive amino and hydroxyl groups in natural nucleoside triphosphates with non-reactive protecting groups. This allows for the formation of 3’-5’ phosphodiester bonds and subsequent deprotection, while minimizing the formation of 2’-5’ phosphodiester bonds and other side reactions. Following synthetic processes and purification steps, highly pure short nucleic acid sequences can be obtained. However, the current chemical synthesis technology for circular RNA can only generate circular RNAs with lengths of fewer than 70-80 nucleotides (nt).
Enzymatic Ligation Synthesis of Circular RNA
Enzymatic ligation for circular RNA synthesis is typically achieved through in vitro transcription (IVT) reactions. This reaction requires a DNA template, reaction buffer, and bacteriophage RNA polymerase. Bacteriophage RNA polymerases are commonly derived from T7, SP6, or T3 phages, with T7 RNA polymerase being the more prevalent choice. IVT reactions enable the cost-effective production of longer circular RNA molecules, making enzymatic synthesis the current mainstream method for circular RNA synthesis. Enzymatic ligation can be further categorized into T4 ligase-mediated ligation and ribozyme-mediated ligation.
T4 Ligase for Circular RNA Synthesis
Various T4 ligases derived from bacteriophages can catalyze RNA ligation reactions, including T4 DNA ligase (T4 Dnl), T4 RNA ligase 1 (T4 Rnl 1), and T4 RNA ligase 2 (T4 Rnl 2). However, this ligation process requires the acceptor substrate on the linear RNA precursor to possess a 3'-OH group, and the donor substrate to have a 5'-monophosphate, in order to proceed.
T4 Dnl and T4 Rnl 1 are suitable for circularizing RNA without complex secondary structures, while T4 Rnl 2 is more appropriate for linear RNA precursors with double-stranded adapter regions. Therefore, in practical applications, it is necessary to choose different T4 ligases based on the secondary structure of the linear RNA precursor. It should be noted that all of these ligation methods cannot achieve circularization of large RNA fragments and cannot completely avoid side reactions associated with intermolecular end ligation.
Nuclease for Circular RNA Synthesis
1) Group I intron self-splicing system
In 1982, scientists discovered the first type I intron within the rRNA transcript of the ciliate Tetrahymena thermophila. This intron also marked the first identification of a ribozyme, an enzyme capable of self-splicing primary transcript RNA without the assistance of any proteins. Since then, numerous self-splicing type I introns have been found in tRNA, mRNA, and rRNA sequences across various organisms and viral genomes. Despite arising from completely different primary transcripts, these type I introns exhibit strikingly similar catalytic core structures. Based on their secondary structure, type I introns can be divided into ten domains, designated as P1 through P10. The catalytic core is located at the junction of the P4-P6 (P4, P5, P6) and P3-P9 (P3, P7, P8, P9) domains. P1 and P10 represent the 5' and 3' splice sites, respectively, while the surrounding sequences around the conserved catalytic core are responsible for maintaining the stability and correct folding of the ribozyme.
The most common method for in vitro circular RNA synthesis currently is the system engineered by harnessing the self-splicing characteristics of type I introns. This approach, also known as the PIE method (permuted introns and exons), relies on the inherent ability of type I introns to self-splice. It requires only GTP and Mg2+ ions to achieve RNA ligation. The in vitro transcription templates commonly employed for PIE synthesis usually incorporate type I intron sequences derived from the Anabaena tRNALeu gene or the thymidylate synthase (td) gene of T4 bacteriophage. Both of these type I introns have been verified to efficiently facilitate RNA circularization.
Compared to chemical and enzymatic methods, the PIE approach enables circularization of larger linear RNA precursors, and its reaction conditions and purification methods are relatively straightforward. Current research has shown that efficient circularization of almost any sequence of linear RNA precursor can be achieved by designing specific PIE transcription templates. Due to these advantages, the PIE method stands as the most extensively studied and widely employed RNA ligation technique at present.
2) Group II intron self-splicing system
Group II introns are a type of mobile genetic element found in bacterial and organellar genomes. They are considered ancestors of spliceosomal introns and retrotransposons in eukaryotes. Group II introns consist of a catalytically active intron RNA and an intron-encoded protein (IEP), working together to facilitate their proliferation within genomes. The intron RNA of Group II introns catalyzes its own splicing through a transesterification reaction similar to spliceosomal introns, resulting in spliced exons and a released intron in the form of a lariat RNA. The IEP, a multifunctional reverse transcriptase (RT), assists in splicing by stabilizing catalytically active RNA structures. Following splicing, the IEP remains associated with the excised intron RNA, forming a ribonucleoprotein (RNP) complex capable of invading the genomic DNA. Group II introns, discovered in bacterial and organellar genomes, are regarded as precursors of spliceosomal introns and retrotransposons in eukaryotes. They comprise a catalytically active intron RNA ("ribozyme") and an intron-encoded protein (IEP), both cooperating to mediate intron proliferation within genomes. Group II intron RNA catalyzes its own splicing via a transesterification reaction akin to spliceosomal introns, yielding spliced exons and a liberated intron in the form of a lariat RNA. The IEP is a versatile reverse transcriptase (RT) that aids splicing by stabilizing catalytically active RNA structures. Post-splicing, the IEP remains associated with the excised intron RNA, forming an ribonucleoprotein (RNP) capable of genome DNA invasion.
Researchers have developed another in vitro circular RNA synthesis technique based on the self-catalytic properties of Group II introns—utilizing the inverse splicing reaction to connect the 5' splice site of one RNA end with the 3' splice site of the same RNA, thereby forming a circular RNA.
3) Hairpin ribozyme
Hairpin ribozymes (HPRs) can generate circular RNA through rolling circle and self-splicing reactions using single-stranded circular DNA as a template. Linear RNA precursors containing HPR will fold into two conformations with cleavage activity, exposing the 3' and 5' ends. These ends then spontaneously join to form circular RNA. While this method efficiently produces shorter circular RNAs, its drawback lies in the fact that the HPR-catalyzed cleavage and ligation represent a dynamic equilibrium, resulting in the instability of the formed circular RNA. Moreover, the generated circular RNA will contain HPR sequences, which could have detrimental effects on its functionality."
Circular RNA Synthesis Method In Vivo
Unlike the in vitro synthesis of circular RNA, the in vivo synthesis of circular RNA is primarily achieved by overexpressing plasmid vectors containing mini-gene sequences that facilitate circularization within living cells. The sequence intended for circularization undergoes natural circularization through reverse splicing reactions following transcription. Most mini-genes for in vivo RNA circularization encompass at least one exon sequence earmarked for circularization, along with intronic cis-elements at the 5' and 3' ends containing splicing elements. By employing diverse combinations of intron-based cis-elements, it is feasible to circularize RNA sequences of nearly any length, ranging from 100 nucleotides to 5 kilobases. These intron-based cis-elements can be generally categorized as intronic complementary sequences, introns containing specific protein binding sites, introns exhibiting ribozyme activity, and introns originating from metazoan tRNAs.
Intronic Complementary Sequences for Circular RNA Synthesis
Based on the existing high-throughput transcriptome sequencing results, it is evident that the majority of endogenous circular RNAs contain sequences rich in reverse complementary motifs on both sides of their introns. These reverse complementary intronic sequences, located on the flanking introns, bring the upstream receptor and downstream donor splice sites of the circularization sequence closer in spatial distance through complementary base pairing, thereby facilitating the occurrence of reverse splicing reactions. The vast majority of intronic complementary sequences (ICS) are derived from repetitive or non-repetitive segments of endogenous genes in organisms. Additionally, a small portion of ICS has been generated through computer simulation design. Currently, researchers have been able to utilize this endogenous circular RNA formation mechanism to prepare specified RNA sequences into circular RNAs.
Specific Protein Binding Sites for Circular RNA Synthesis
Various RNA binding proteins (RBPs) play a role in promoting reverse splicing within organisms. Therefore, we can incorporate the binding sites of such proteins into overexpressed circularization vectors to mediate the circularization of target RNAs. In addition to RBPs, other proteins such as NF90/110, RBM20, Fus, DHX9, can regulate the intracellular synthesis of circular RNAs.
tRNA Introns for Circular RNA Synthesis
A class of abundant circular RNAs derived from tRNA introns, known as tRNA intron circular RNAs (tricRNAs), can be produced independently of spliceosomes and self-catalytic splicing reactions. They are primarily generated through the cleavage of tRNA precursor molecules containing introns by a set of highly conserved host enzymes. Subsequently, the excised introns are internally ligated by the host ligase RctB to form circular RNA molecules.
Prospects of Circular RNA Synthesis
Circular RNA, owing to its remarkable stability, holds the potential to serve as an effective and safe next-generation vaccine delivery platform. Previously, the artificial synthesis of circular RNA largely relied on in vitro strategies based on biochemistry. However, in recent years, novel molecular biology-based methods have emerged, enabling the direct production of circular RNA within living cells. It is conceivable that in the future, more refined and standardized methods for the fabrication of artificial circular RNA will emerge. These advanced circular RNA preparation techniques are bound to significantly propel the research progress in the field of circular RNA biology, functions, and molecular mechanisms.
References
1. Chen, X.; et al. Circular RNA: Biosynthesis in Vitro. Frontiers in Bioengineering and Biotechnology. 2021, 9.
2. Obi P.; et al. The Design and Synthesis Of Circular RNAs. Methods. 2021(196): 85-103.